Exploring the Early Universe with ALICE: Insights into QGP
Written on
Chapter 1: Unveiling the Early Universe
The ALICE collaboration at CERN has made significant strides in understanding the early universe by measuring the flow of bottomonium particles for the first time. This discovery sheds light on the Quark-Gluon Plasma (QGP) that existed in the universe's earliest moments.
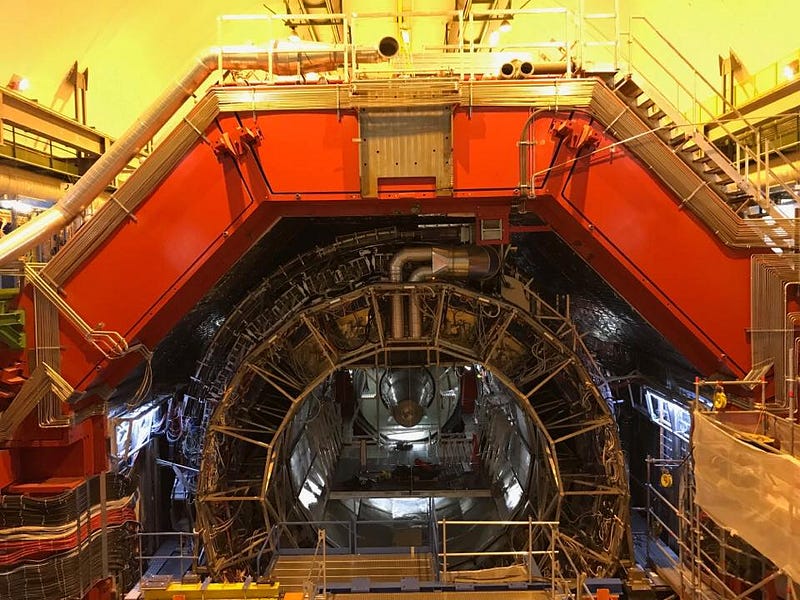
The ALICE detector, an advanced scientific apparatus at CERN, investigates early universe conditions by creating quark-gluon plasma and analyzing the particles that emerge from this state. During a presentation at the European Physical Society's conference on high-energy physics, the ALICE collaboration reported their groundbreaking first measurement of the flow of bottomonium—a heavy meson particle.
The analysis of bottomonium particles, a form of upsilon particle, is crucial for understanding the QGP that filled the universe just a few millionths of a second after the Big Bang. By monitoring pairs of muons—heavy electrons that result from bottomonium decay—the team found that these particles exhibit unexpectedly low elliptic flow, which is an indicator of how energy and momentum are distributed among particles from the perspective of the beamline.
This finding contrasts with previous studies of other hadrons, which displayed considerable elliptic flow.
David Evans, a professor of high-energy physics at the University of Birmingham and a key figure in the UK’s participation in ALICE, remarked, “Elliptic flow measurements in ALICE indicate that the Quark-Gluon Plasma behaves like an almost perfect liquid, with light quarks (up, down, strange, and charm) flowing uniformly with the system.”
The absence of significant elliptic flow in bottomonium suggests that b-quarks are produced solely during the initial collision of lead ions, prior to the formation of the QGP. These results align with established theories positing that upsilon particles do not move along with other particles as the plasma expands, providing researchers with deeper insights into the conditions of the universe's earliest moments.
Professor Evans further elaborated, “This makes b-quarks and their composites ideal probes for studying the initial phases of the QGP, as they undergo the entire evolution of the system.”
The first video, titled "A New Era for Emission-line Probes of Star-forming Galaxies back to Cosmic..." by Alice Shapley (UCLA), explores advanced methods in examining star-forming galaxies, which complements our understanding of the universe's early conditions.
Chapter 2: Understanding Bottomonium
The journey through the 'particle zoo' reveals the nature and significance of bottomonium particles in the context of the ALICE experiment.
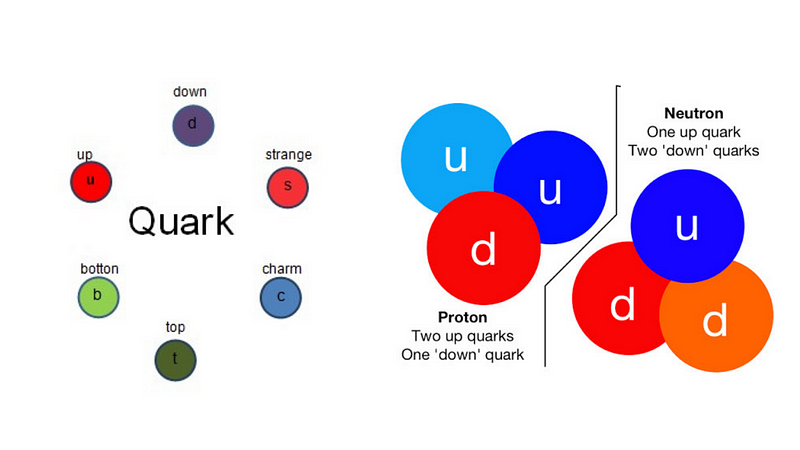
Bottomonium is classified as a heavy meson, consisting of a bottom quark (b-quark) and its antiparticle. These subatomic entities are highly unstable, existing only for brief periods at elevated energy levels before decaying into other particles. When bottomonium decays, it produces pairs of muons.
Formed during high-energy lead-lead collisions at the LHC, bottomonium particles act as excellent probes of the QGP that existed mere moments after the Big Bang. Their production at the start of collision events allows them to experience the plasma's entire evolution, from its formation to the cooling phase where hadrons can emerge.
This early epoch in the universe's history was unique, as quarks and gluons existed freely in plasma instead of being confined within protons, neutrons, and other hadrons. The extreme temperatures of that time permitted this free existence, as quarks and gluons are not observed as free particles in our current epoch.
Recreating such conditions requires immense energy, which the Large Hadron Collider (LHC) provides. As the world's most powerful particle accelerator, the LHC achieves collisions that generate temperatures approximately 400,000 times hotter than the sun's core, melting protons and neutrons and liberating quarks and gluons to form a QGP.
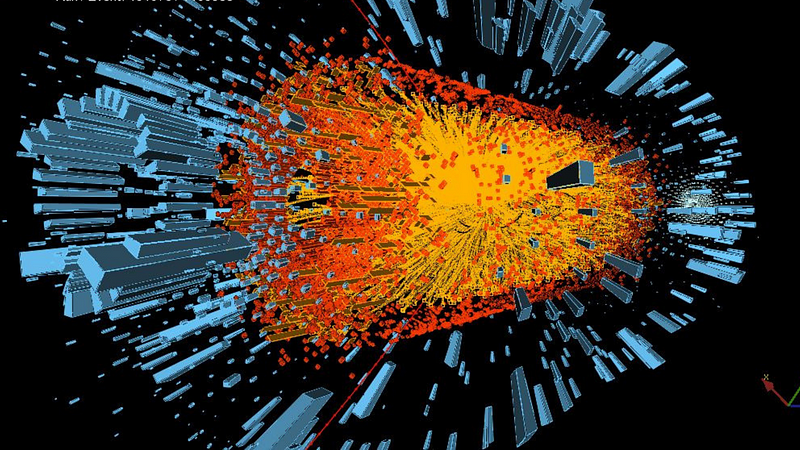
The ALICE collaboration, comprising over 1,000 scientists, operates a massive 10,000-ton, 16-meter tall detector located 56 meters underground in the Jura mountains, aiming to recreate these high-energy states by colliding lead ions instead of protons, as seen in other LHC experiments.
The advantage of lead-ion collisions lies in their abundance of quarks, enhancing the likelihood of significant observations. While a proton and neutron each contain three quarks, lead ions comprise at least 56 protons and 204 neutrons, providing a richer environment for research.
ALICE measures the QGP as it expands and cools, relying on indirect methods to ascertain the properties and presence of QGP through the signatures left by muon pairs generated from decay. One key signature is elliptic flow, which reflects the collective movement of produced particles influenced by factors such as type, mass, collision angle, and momentum—this flow arises from the hot plasma's expansion following lead-ion collisions.
The second video, "Simona Procacci: Minicourse on Gravitational Wave Probes of the Early Universe - Class 1," delves into gravitational wave research, offering additional perspectives on early universe studies.
Chapter 3: Future Prospects for ALICE
The most exciting aspect of ALICE's efforts to investigate the early universe is the upcoming high-luminosity upgrade, which promises to significantly enhance the data available for researchers.
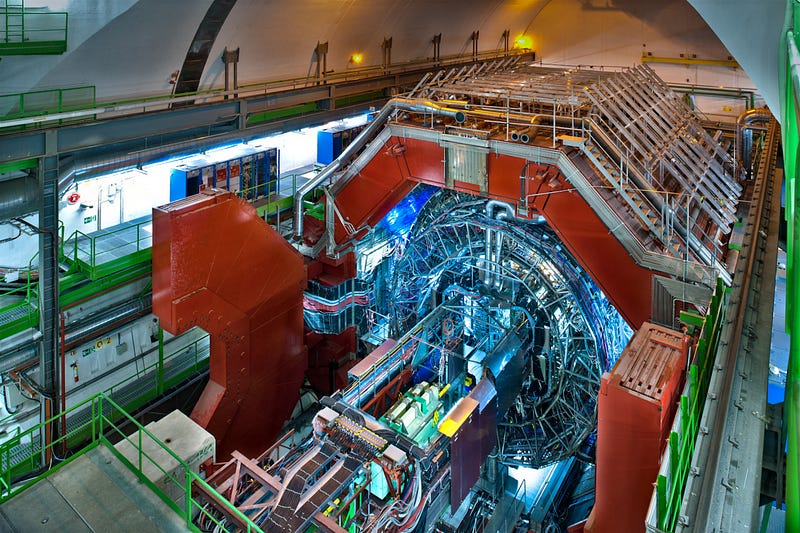
CERN anticipates that this upgrade will be operational by 2021, aiming to increase the luminosity of the LHC by a factor of 10. In particle accelerator terms, luminosity correlates with collision frequency, meaning that a higher luminosity leads to more collisions.
For context, while the LHC produced 3 million Higgs boson particles in 2017, the High-Luminosity LHC is projected to yield 15 million annually. Along with the high-luminosity upgrade initiated in 2018, ALICE will undergo various enhancements, resulting in an expected overall data increase of 100 times current levels.
Evans expresses optimism about these advancements: “With this substantial increase in statistics and a new inner detector, we will measure b-quark particles with greater precision.”
The experiment's enhancements offer substantial potential for improving our understanding of the QGP and the conditions of the early universe, with recent bottomonium findings paving the way for future discoveries. Evans concludes, “[The upgrades] enable us to investigate the properties of the QGP in much greater depth, thereby expanding our knowledge of the universe's early evolution.”